Origin of the Universe
There
are several theories for the origin of the universe. Some of the popular ideas
have one significant postulate that the universe originated ex-nihilo. It means
that the universe is created 'out of nothing.' We will analyze this concept
based on scientific methods to find the truth. The modern Big Bang theory of
the universe is the most accepted theory based on scientific concepts. It can
explain several phenomena in the universe, such as the galaxies' origin and the
planetary systems' formation.
The
composition of various universe's constituents can provide pointers for its
origination. Also, the constituents' composition reveals the universe's four
fundamental interactions. Understanding the relationship of these interactions
with the physical entities points to features of the universe that are the operating
principles for the various phenomenon happening in the universe. Therefore, we
will present the Big Bang theory of the universe's origin based on the
constituents' composition and characteristics.
Characteristics of the constituents
We
know that matter, energy, dark matter, and dark energy constitute the universe.
The composition and fundamental interaction of the constituents provide
indicators for the universe's origination. Therefore, we present the
fundamental particles and interactions essential for understanding the universe's
origin.
Matter
and antimatter
The
gravitational interaction holds all the planets in orbits around a star.
Similarly, the stars are bound to galaxies. The gravitational
interaction is responsible for maintaining the galaxies and the planets
together. The amount of matter in a planet or anything is measured by the mass
of a body. Any two bodies made of matter have a mutual gravitational force of attraction.
The gravitational interaction between two bodies is proportional to the masses
of the bodies. The constant of proportionality is known as the gravitational
constant. It is also one of the universal constants.
All
galaxies, stars, and planets are made of matter. Matter
is made of atoms that have several elementary particles. A simplified diagram
of an atom is shown in Figure
1 21.
As shown in Figure 1.21, the nucleus of an atom is made of neutrons and protons.
The protons or neutrons are made of quarks. There are many more such elementary
particles that make up an atom.
Figure 1 21:
A simplified diagram illustrates an atom's structure (not to scale, the nucleus
is much smaller in an atom).
All
the elementary particles are grouped into two categories as fermions and
bosons. The fermions are also known as matter particles, while the bosons are interaction
carriers. One well-known example of a boson is the photons we see as light. The
other types of bosons help in gluing the fermions together. Thus, the gluons pack
protons and neutrons in the nucleus of an atom. Furthermore, each of the matter
particles has an antimatter particle. Therefore, antimatter exists, although we
observe it mainly in laboratories.
The
protons and neutrons in the nucleus are held together with a powerful force
called strong interaction. It is a fundamental interaction. In addition, one
more fundamental interaction in an atom is known as the weak interaction. Radioactive
decay and nuclear fission are possible due to weak interaction.
Energy
Various
types of energy exist in the universe. For example, we get heat and light
radiation energy from the Sun. Also, the radiation energy in the interstellar
medium is an example of electromagnetic waves. Electromagnetic waves exert a
force known as electromagnetic interaction. It is another fundamental
interaction, and photons are the carrier of it. The energy of an electromagnetic
wave is proportional to its frequency. Therefore, we can use a constant to
convert the frequency into energy for an electromagnetic wave. We call it Planck's
constant, and it is also a universal constant.
As
mentioned earlier, light is also a form of electromagnetic wave. The velocity
of electromagnetic waves is constant and equal to the velocity of light. Moreover,
it is constant everywhere in the universe. Thus, the velocity of light is also
a universal constant.
Dark
matter
Dark
matter is not visible as it does not interact with ordinary matter and energy.
However, it exerts a considerable gravitational interaction indicating that it
is made of something very dense. Therefore, dark matter may have
black holes. A black hole is a region in spacetime with intense gravity so that
even light cannot escape from it.
Dark
energy
Matter,
energy, and dark matter exist in the universe's space. Even the existing space is
a form of energy. It is known as dark or vacuum energy. Dark energy has a
unique property in that it exerts negative pressure. Thus, it causes the
expansion of the universe. Dark energy is the most significant constituent of
the universe.
From
the composition of the four constituents of the universe, we learn about four
fundamental interactions. Also, we find three universal constants used to
represent the relationships of interactions with the components. We
can express the relationship between matter, energy, and interactions by
equations involving a few constants. These constants hold their value everywhere
in the universe. The universal constants are the gravitational constant G,
the velocity of light c, and the Planck constant ℏ.
Mass to
energy conversion
It
is well known that matter and energy are interconvertible. The conversion of
matter of mass 'm' to energy 'E' is
governed by the equation
E=mc2
where
'c' is the velocity of light. Thus, matter can be created from energy or vice
versa.
It
is also possible to create matter from space or vacuum energy. We can explain
this from the uncertainty principle. We know that it is impossible to measure the position
and momentum of any matter particle with arbitrary accuracy. It is known as the
uncertainty principle. For example, to measure the velocity of a quark along
with its position, light or anything used to measure it will change the
position itself. Such changes are known as quantum fluctuations. Due
to quantum fluctuations, elementary particles and antiparticles are generated
and annihilated continuously in a vacuum. These elementary
particles constitute matter.
It
is also possible to generate energy by combining the elementary particle and
antiparticle. Thus, matter and antimatter annihilate to create energy. For
example, Figure
1 22
depicts the annihilation of two matter particles, an electron and a positron.
The annihilation produces two gamma rays as energy.
Figure 1 22:
An illustration of matter particles electron and positron annihilating to two
gamma rays' energy.
Now, we are familiar with the essential attributes of the
components and the universe. Therefore, we can postulate a theory of the origin
of the universe.
The Big Bang Universe
We
will briefly present the Big Bang theory of the universe's origin. After that,
we will explain the creation of all the constituents of the universe.
As
mentioned
earlier, gravitational forces hold the planetary systems and galaxies together.
On the other hand, we observe that the galaxies are moving away from each
other. Using modern tools and techniques, we can measure the recession
velocities of the galaxies. From any observation point, some galaxies will be
nearby while others will be relatively far. The velocities of the galaxies are
proportional to their distance from the observation point. The galaxies
farthest away are moving with the greatest speed. This observation implies that
all the galaxies would have been together if we extrapolate backward in time.
In the distant past, these started moving away from a single point.
At
the beginning of the universe, there was no time and no matter except this
single point. It is postulated that the whole mass of the universe was
concentrated in the point; it had almost infinite density and temperature, and
this starting point is called the gravitational 'singularity.' This point
started expanding rapidly in a kind of burst. This proposition about the universe's
origin is known as the Big Bang theory. An illustration of a few events in the
universe after the Big Bang is shown in Figure
1 23.
Figure
1 23:
It illustrates the formation of elementary particles and the first hydrogen
element in the events after the Big Bang (not to the scale).
As
shown in Figure
1 23,
at
the start of the universe time, 10−43
second,
the temperature
was above 1032 degrees Kelvin. The
expansion of the universe results in the fall of temperature in space.
Within a tiny fraction of a second after starting, the expansion became more
rapid. Following the exponential inflation, the conditions became conducive to producing
the elementary particles of the atoms within a fraction of the first second. The
temperature was favorable for creating elementary matter particles and
antiparticles. All the space became occupied by a soup of hot ionized
quark-gluon plasma. About one second after the Big Bang, it was
still very hot at about 10 billion degrees Kelvin. The universe was a big sea
of electrons, protons, neutrons, photons, positrons and neutrinos.
As
it continued to cool down to about a billion degrees at about 10 seconds, the
protons and the neutrons started to combine to make deuterium. After that,
atoms began to form as electrons combined with deuterium to make hydrogen and
helium in a process known as Big Bang nucleosynthesis, as shown in Figure
1 23.
Before
these combinations, the universe was opaque due to elementary particles all over
space. However, the combination process created empty spaces, and the universe
became transparent. The combination process happened between 375,000 to 380,000
years after the Big Bang when the universe's temperature dropped to
about 4000 degrees Kelvin. This process of
recombination continued for nearly 100 years. We know that the photons are
emitted during the recombination process. The photons can move freely as the
combination also creates voids. As a result, the universe becomes transparent.
These radiations are observed as cosmic microwave background radiation (CMBR)
presently. It is also referred to as the afterglow of the Big
Bang that can be seen today.
As
shown in the Figure 1.21, hydrogen and helium are the oldest elements formed
from elementary particles. Lithium and beryllium were constituted from hydrogen
and helium as the conditions of temperature and pressure were favorable for
such formations. However, the conditions were not conducive to creating still
heavier elements.
The
big blobs of hydrogen and helium gas were the initial stages of the stars.
Later, the fusion reaction formed heavier elements in the different layers of
these stars. The fusion reactions are exothermic reactions that give out heat
as it is happening in the Sun. The formation of heavier elements up to iron
happens by fusion. Still heavier elements are formed by proton or neutron
capture in the stellar systems.
In
the early stages of the universe, with the element formation, the atoms accumulated
due to gravity. As a result, we observe most of the material mass is
concentrated in the galaxies. However, the galaxies also have enormous giant
molecular clouds that are very dynamic due to the stellar winds. The stellar
winds are fast-moving flow flows of material such as protons, electrons, and
atoms of heavier metals that are ejected from stars.
At
some point, the molecules start to collapse into each other due to gravity. It
becomes a catastrophic event with a rotator motion, acquiring more mass with
each rotation. This process is known as accretion resulting in a star. As the
accretion disc rotates, not all the mass is accreted by the star itself. Instead,
there are also other accretion points revolving around the disc, and these
become the star's planets.
A
representation of the timeline of the Big Bang universe is shown in Figure
1 24.
As shown in Figure 1.24, the universe originated from a point that started
expanding. Then, it created matter particles due to quantum fluctuation. It is
followed by rapid inflation resulting in the cooling of the universe. Finally,
it created a condition for recombination that gave to afterglow light seen as
CMBR. The first star appeared at about 400 million years after Big Bang. After
that formation of galaxies happened, giving rise to the present universe.
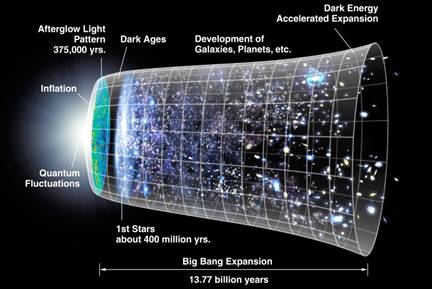
Figure 1 24:
An illustration depicting the timeline for the significant events of the
universe beginning from the Big Bang.
Source: Credit to NASA/WMAP
Science Team [Public domain].
In
the present universe, we have matter and energy. In addition, there are two
other components: dark energy and dark matter. Dark matter is a matter that we
cannot see, but it exerts considerable gravitational influence. Dark energy is
a type of energy causing the universe's expansion. It is the most significant
component of the universe. It is estimated that dark energy is 69% of all the
matter-energy composition of the universe. The second major universe component
is dark matter (26%). The rest of the universe is constituted by baryonic
matter and less than 5% radiations.
The
density of matter-energy in the universe plays a role in determining the universe's
shape. Computer simulation shows different shapes of the universe depending on
the density. A density that makes the universe flat and infinite is known as
critical density. At present, it is estimated that the matter-energy density of
the universe is equal to the critical density, which implies that we have a
flat universe. The flat universe means that it will continue to expand forever.
However, suppose the density ever becomes greater than the critical density. In
that case, the expansion will reach maximum, and it will start to slow down
from there, ultimately leading to a big crunch. The big crunch is one
possible scenario in which the universe's expansion eventually reverses, and
the universe collapses to size 'zero' again.
The
universe's age is estimated based on the galaxies' measured velocities. The
estimate predicts the age of the universe to be 13.8 billion years. The age has
also been verified based on the parameters of a few other natural phenomena.
All the evidence supports the universe's age to be 13.8 billion years with an
accuracy of plus or minus 21 million years.
The
earliest traces of life on Earth are found in the imprints of microbes
preserved in sedimentary rocks estimated to be more than 3.8 billion years old.
Carbon, hydrogen, oxygen, and nitrogen are the major constituents of all living
bodies. Initially, these individual elements were formed in atomic forms. Then,
a specific combination of the atomic elements created a molecular state. Finally,
these molecules combined further with a particular arrangement to form a long
chain. One of these long chains that we recognize as deoxyribonucleic acid
(DNA) was the seed for the emergence of life.
When
the conditions were ripe for the emergence of life, the evolution of living
beings started. The evolution from single-cell organisms to multi-cell complex
animals and plants is well-established. With evolution came intelligent life,
especially in the form of human beings. The curiosity of human beings started
the search for finding the origins.